Your cart is currently empty!
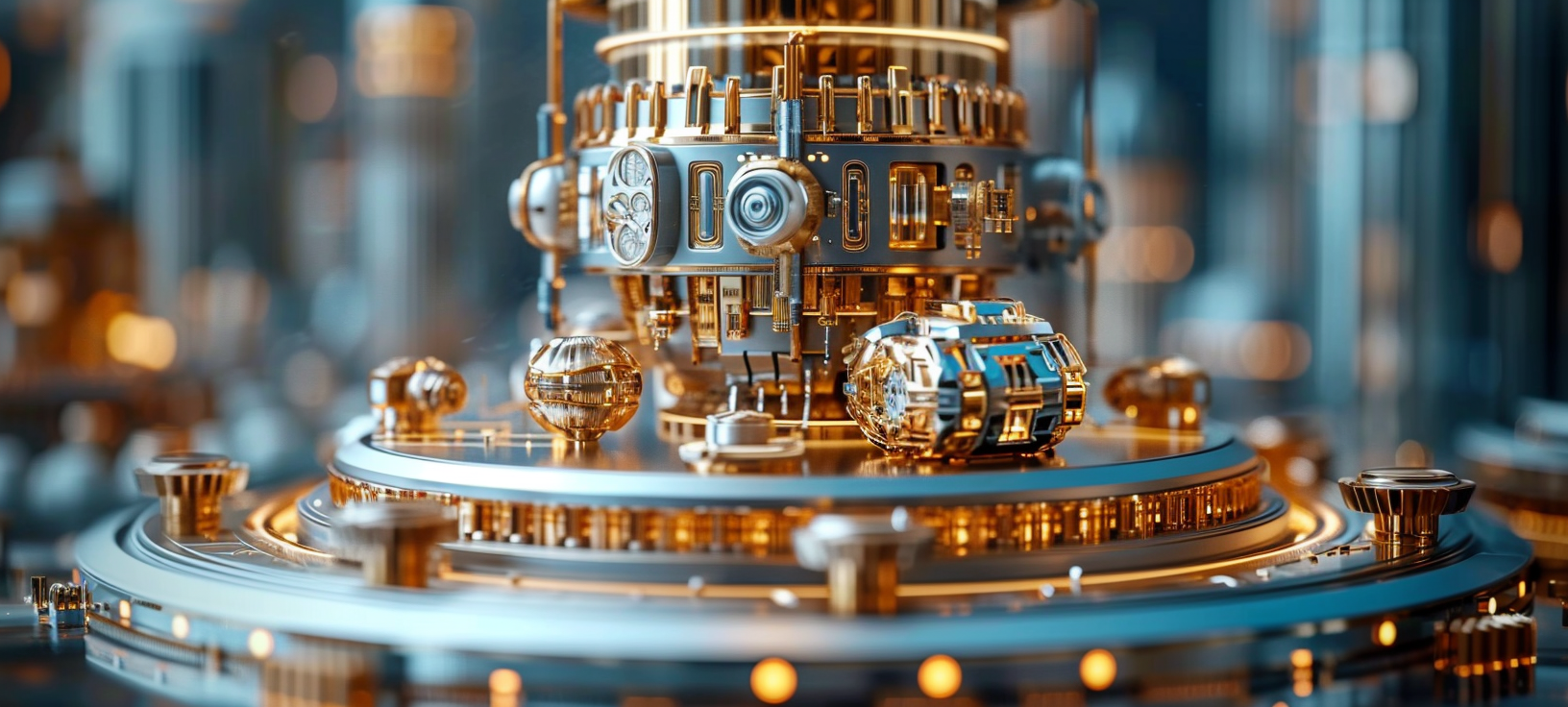
What is Quantum Computing?
Introduction to Quantum Computing

You are only familiar with one information processing model: ordinary classical computers. However, quantum computing promises to change all that by taking advantage of the laws of quantum mechanics to achieve new feats of speed and complexity beyond what is possible through conventional means. In a classic computer, the fundamental unit of information is the binary digit, or ‘bit,’ represented by two states – 0 and 1. (Correspondingly, a group of eight bits is known as a byte.) In a quantum computer, the fundamental unit of information is the quantum bit, or ‘qubit,’ which combines multiple states simultaneously via a quantum mechanical effect known as superposition – a phenomenon with no classical equivalent. Therefore, a prototype quantum computer can process millions of possibilities in parallel.
It wasn’t until the mid-20th century. However, these concepts were formalized into quantum mechanics, the branch of physics that describes how particles on the microscopic scale behave. The first theoretical description of how such a machine might work didn’t appear until the 1980s. According to conventional physics, the most a computer can compute in a single step is the product of two numbers. For example, a quantum-version version of a multiplication gate could produce the sum of those numbers on quantum computers.
Quantum computers can promise solutions to problems that are intractable with classical computers. From drug design and material science to optimization problems and cryptography, no area can escape the impact of quantum computing, which will swiftly transform many industries and scientific fields.
Understanding quantum computing requires first understanding quantum mechanics, the basic principles that make quantum computers work; that is why I have devoted the first half of the book to the essentials of quantum mechanics: what is meant by superposition, entanglement, and quantum interference, and why that allows quantum computers to do things that classical computers do not.
You’ve just taken the first step to becoming an informed citizen of the quantum world. From here, you can take a more in-depth look at the basics of quantum computing, the technologies behind it, where it is applicable, and where it falls short of delivering the promise it has long offered. As we decipher the quantum domain, we start to comprehend the potential of a technology that lies at the forefront of the next wave of computing, offering to reshape what can be done computationally.
Fundamentals of Quantum Computing
A quantum computer is based on several new principles that are very different from those of classical computing. Without them, the possibilities of quantum computing would not exist. Understanding these principles is the key to understanding what quantum computers can do.
The crux of quantum computing is its quantum bit, usually evidenced by using the ‘qubit’ – or quantum bit. Instead of having a binary outcome, where a qubit can be either a zero or a one, a classical bit’s macroscopic particles carry the extra information – through the particle’s polarisation, spin, vibrational state, and others – on whether it represents a zero or a one. However, a qubit, which carries nano dimensions, is constructed not from the concept of symmetry, as in a classical circuit, but from quantum mechanical superposition, and that’s what makes this nanoscopic bit much more powerful. Here’s what this entails. A qubit can not only carry a zero and a one, but it can also be in a superposition of both states – think parallel universes, your wavefunction split between you if you’re after a movie, or your cat dead and alive.
Quantum Entanglement: Another pillar of quantum computing is entanglement, a quantum phenomenon in which two particles (no matter the distance from each other) get connected so the state of one can instantly alter the state of the other, creating a potent connection between the qubits of a quantum computer that allows it to do massively complex calculations more efficiently and in a shorter amount of time than an ordinary (or classical) computer.
Quantum Gates and Circuits: In classical computing, we take bits and process their logical functions with logic gates. Qubits are also processed in quantum computing using gates that control their states by quantum mechanics. Since quantum bits interfere with each other, as we discussed earlier, this means that quantum circuits process information fundamentally differently from their classical counterparts.
Across several regions of his mind, there hovered countless possibilities and potentials in the form of quantum amplitudes. With each four-faced crucial roll between Meer and Blass, his mind became an exponentially expanding analog computer that updated itself more quickly each time. Adding, multiplying, amplifying, and canceling out these amplitudes started the back-and-forth of the wave function, which is the hallmark of quantum computation. The probability of some particular sequence of results was, in some places, amplified and, in others, canceled out. As such, the quantum algorithm could move along hundreds or thousands of paths from the start of the game to its conclusion. The critical step was interference, where quantum amplitudes were added up, multiplying some computation paths and eliminating others in directional coherence. Without this principle, quantum computation could achieve no speed-ups over classical computation.
Learning these basics can start to make the unique capabilities of quantum computing clear and show why it could solve problems that classical computers can’t. Striking at the heart of this computational divide are the concepts of superposition and entanglement. With a superposition, a quantum computer can explore far more of a given computational space simultaneously than a classical computer can, and by extending that capability to multiple entangled qubits, the computational possibilities multiply. This unique advantage might seem dim, but quantum computers could enable us to do things that traditional computing can’t, from more robust kinds of cryptography to vastly improved modeling of the most complicated systems and beyond – providing a once-in-a-generation leap in computing power.
Comparing Classical and Quantum Computing
The differences between classical and quantum computing are rooted in the philosophies of the two technologies. There are different ways of thinking about the concept of information, and it is essential to consider and appreciate how quantum computing is changing everything.
Speed and Efficiency: One of the most noticeable differences is the speed or efficiency that quantum computers may one day have. Because quantum computers take advantage of quantum superposition and entanglement to process logical functions, they can theoretically accomplish processing at speed faster than any classical computer. Classical computers process information one at a time in a linear fashion. Quantum computers, as prototype systems have demonstrated, can process up to thousands of possibilities simultaneously (in a superposition state).
Computational Abilities: Classical computers are currently orders of magnitude faster and more capable than quantum computers, meaning they can solve a broader range of tasks. However, for some computational issues involving searching enormous spaces of potential solutions or simulating quantum mechanical systems, the vast parallelism in quantum computers can quickly evaluate millions of outcomes. Superposition vastly increases our ability to sample possible solutions concurrently.
Use Cases: Classical computing is helpful for everyday activities, from running word processors and internet and app access to business operations and databases. Quantum computing is designed for specific complex tasks that present virtually impenetrable challenges to classical computing: simulation of huge systems and properties of molecules, massive optimization for complex entities and problems, and brute-force cryptography.
It’s far from a competition between quantum and classical computing: they are approaching a complementary relationship in which each will excel in different applications, be robust in specific ways that the other isn’t, and play to different strengths. Most computations will benefit from a hybrid quantum-classical approach. In the foreseeable future, any progress with practical quantum computers will operate with classical computers, creating vastly enhanced computational power and a suite of new problems for science and industry to tackle.
Technological Advances in Quantum
However, these technological advances brought us closer to the reality of quantum computing, helping to convert abstract ideas into practical possibilities.
Advances and Novel Contributions: In recent years, there have been breakthroughs in quantum computing, such as more stable qubits, quantum error correction, and quantum supremacy in solving problems faster than the best classical computers.
Quantum processors and machines: The history of quantum processors involves the number of qubits, a term that refers to the number of quantum states of a particle. Quantum processors have multiple qubits, and people worldwide are working to amass more and more qubits to enhance the power of their computers. Google, IBM, and D-Wave are among the companies that have built available quantum processors. The D-Wave 2000Q processor has 2000 qubits. Currently, these processors are used in quantum machines designed to solve problems that classical computers using classical processors cannot resolve.
Scalability and Integration: Quantum computing’s potential can be fully realized only if it becomes scalable and if an ever-growing number of qubits can be inserted into quantum computers without compromising their stability. Hybrid architectures are designed to merge quantum computers with classical systems to develop a hybrid computational framework.
But quantum advances are not just increases in computational speed or brute force—they’re also improvements that make the technology more usable, more accessible, and easier to design laboratory and industrial applications. Given that quantum research and development are accelerating, expect to see more significant numbers, brighter colors, and more uses.

Quantum Computing Applications
Quantum computing promises to solve problems intractable for classical computers in fields ranging from cryptography to quantum chemistry, machine learning, and logistics routing. This is possible because of the unique way in which it manipulates information.
Cryptography: One of the most notorious uses of a quantum computer is cryptography. Quantum computers can break many of the cryptographic systems that are currently used. Similarly, we need to develop quantum-resistant encryption schemes that are secure against attacks from quantum computers. One of modern human history’s most significant arms races is quantum computing power versus cryptographic security.
Drug discovery: One of the most prominent use cases for quantum computing is in the pharmaceutical industry, which can potentially reduce the time and cost of developing new drugs. For example, it can simulate molecular interactions, potentially delivering more target-specific medicines faster.
Artificial Intelligence: Real-world applications entail the potential to enhance artificial intelligence (AI) in processing complex data sets or performing calculations, something that classical computers can’t do given their classical physics limitations. Mind-melding or mixing up quantum computing and AI may accelerate machine learning for more intelligent and efficient AI systems.
Optimization Problems: Companies dealing with logistics, supply chain management, energy distribution, and traffic optimization face complex optimization problems; quantum computing can process and analyze them more quickly, deriving solutions that optimize resources and, thus, costs.
Quantum computing has numerous applications and could significantly impact different sectors. For example, quantum computers can solve certain kinds of simulations and calculations at an unprecedented speed, leading to innovation and optimization in various industries.
Challenges in Quantum
Though it theoretically promises wonderfully efficient performance beyond our current capabilities, building a quantum computer will require meeting several critical technical and operational challenges – some novel and some more theoretical.
The second obstacle is the technical hurdle of engineering reliable qubits. Qubits are highly sensitive to their environment; even the slightest change in temperature or electromagnetic field can cause the quantum state to be ‘lost’ (a phenomenon known as decoherence). Computationally functional qubits must be able to maintain (or ‘cohere’) quantum states for sufficiently long times.
Scalability: The bigger the quantum computer, the more difficult it is to preserve the quantum state of more qubits and get them all to carry out the programmed instructions. Scientists are now working on improving fault-tolerance using fault-tolerant protocols. Most of our efforts will be focused on identifying a relevant problem and developing a target device to solve it. Since each quantum system – whether based on quantum computing or sensing – involves unique engineering challenges, it is an interesting scientific question why nature ended up with two types of shared quantum resources.
Error Rates: Qubits and the operations made on them are fragile and noisy. Special quantum operations called quantum error correction to detect and correct these errors, which adds extra qubits and makes quantum circuits more complicated. Reducing the error rate and keeping quantum circuits efficient and scalable is a fundamental yet challenging problem for quantum computing.
Quantum Decoherence is the foremost hurdle to making quantum computers. Decoherence describes how qubits lose their quantumness and behave as classical bits due to unwanted environmental interaction. One current goal of researchers is to create ways to isolate qubits from decoherence by preventing them from coupling to their environment in a rapid time frame.
They are further reminders that turning quantum computing into a practical reality will be a challenge – one that will keep quantum physicists, materials scientists, and engineers constantly pushing the boundaries to innovate new solutions. On the other hand, as progress continues to be made in bridging these challenges, hope for a quantum future, fuelled by the many opportunities quantum computing holds for future technology and society, keeps getting stronger.
Future of Quantum Computing
The fantastic quantum computing future ahead of us offers many possibilities and problems. Quantum computing is likely to revolutionize many sectors.
Theoretical predictions: Continuing theoretical advances in quantum computing are starting to push the limits of what is feasible, and it would seem that future quantum computers would be able to solve particular classes of problems in seconds that can take classical computers millennia: simulating large quantum systems, for example, and yielding insights about materials science, chemistry and physics.
Potential impact: across industries. From finances, where quantum computing is expected to improve trading strategies and risk management, to healthcare, which could be used to accelerate drug discovery (especially genetic analysis) and personalized medicine, quantum computing is set to revolutionize vast parts of our lives. Logistics is another area expected to benefit from quantum computers, which could be used to optimize global supply chains to reduce costs and improve efficiency.
Scaling quantum technologies: The ability to scale quantum technologies to build larger, more robust quantum computers is critical for the future and presents a significant challenge. This encompasses the creation of quantum computers with higher numbers of qubits and higher quality, developing scalable quantum error correction technology, and creating scalable architectures that allow the implementation of rich sets of quantum algorithms.
Embedded in classical systems: What is more likely to come is a future in which the two will complement each other. Quantum computing will complement classical computing in hybrid systems designed to harness the unique features of each type of computer, which could give us the fastest possible solutions, where quantum processors work on particular tasks to leverage their distinctive advantages.
Ethical and societal questions: With the advent of more useful quantum computers, we will have to start considering data privacy and the digital divide.
In short, the quantum future represents a vast and uncluttered scientific and technological wilderness ripe with unexplored possibilities. How we walk this brave new world should be guided by a mindset that actively nurtures and cultivates this immense promise for the betterment of humankind.
Quantum and Cybersecurity
Quantum computing and cybersecurity play vital roles in the future of information security, with quantum technologies promising to pose new dangers and provide new solutions.
Two sides of the coin: On the one hand, quantum computing could be a real cybersecurity threat. Quantum algorithms, such as Shor’s over-factoring algorithm, would be capable of ‘solving’ commonly used encryption schemes such as RSA and ECC (Elliptic curve cryptography) and would theoretically allow someone to ‘crack’ the key of any encryption system – so the protection of a ‘vast amount’ of our data could be in danger. On the other hand, quantum computing could also boost the cybersecurity of our systems and data. Thanks to quantum computing, research is being carried out on developing Quantum-resistant encryption algorithms that would be safe and more resilient to quantum attacks.
More generally, the emerging field of quantum-safe cryptography focuses on devising encryption forms that are provably secure against any foreseeable classical or quantum computation attacks. One promising type of quantum-safe cryptography, known as quantum key distribution (QKD), provides a quantum-mechanical method for secure communication that guarantees detection of any attempt to intercept the communication.
The quantum era of cybersecurity is coming or is already upon us. This is now apparent to governments and industries worldwide, who are taking steps towards planning for a future in which they can take advantage of quantum computing, be prepared for it, and make their current cryptographic systems quantum-safe. In some countries, national agencies commission studies of the emerging field of quantum cybersecurity; research funding is available in others, and even NASA is organizing a ‘Quantum Artificial Intelligence Impact workshop.’
Ethical and Legal Challenges: Massive quantum computing in cyberspace has ethical and legal challenges. The moral and legal questions posed by quantum computing and quantum cryptography force us to consider balancing the benefits of advanced quantum cryptographic tools against protecting a civilian population’s right to privacy, data protection, and national security.
In summary, quantum computing is both a threat and a chance for cybersecurity. Two significant challenges, developing quantum-safe encryption and strategic planning for a post-quantum world, explain how the cybersecurity industry can survive the quantum age and keep information assets safe in the increasingly emerging cyber-dependent world.
Aside from those philosophical questions, the capacity to develop faster and more powerful quantum computing is also centered on making the technology feasible and usable. Increased quantum-computing research will inevitably advance the technology’s adaptability. We’ll see a growth of quantum computing applications in pure science, industry, and society.
Quantum Computing Applications
In theory, quantum computing can tackle problems across the technological spectrum that would otherwise be impossible for classical computers.
Cryptography: Quantum computing is essential in cryptography, and quantum computers can quickly break most cryptographic algorithms. So, quantum-resistant encryption is already under construction. It will inevitably face an arms race with the extent of quantum computing power.
Drug Discovery: Quantum computing has the potential to revolutionize drug discovery in the pharmaceutical industry. It can simulate molecular behavior at a quantum level, and by simulating drug interactions at a finer resolution, researchers can optimize drug performance and facilitate faster drug design. This capability dramatically reduces the time and expense of bringing new medications to the market.
Potential impact: 2) Quantum computing can improve artificial intelligence (AI) by processing large data sets and performing calculations beyond the capabilities of a classical computer. The synergy between quantum computing and AI could make machine learning more innovative and efficient.
Complex Optimisation Problems: The industry is rife with complex optimization problems, including multimodal logistics planning and supply chain optimization, energy distribution optimization, and multi-criteria traffic flow optimization. Quantum computing can run and analyze such issues optimally, generating solutions and optimizing resources.
The influences of quantum computing are vast. As a versatile technology that can solve a wide range of simulations and calculations at unrivaled speeds, the application areas of quantum computers are countless.
Challenges in Quantum
This is where the promise of future quantum computers intersects with mid-20th-century material realities. A set of unique technical, operational, and theoretical challenges stand between present hardware and a feasible long-term, practical, and scalable quantum computer.
Technical Hurdles: Building and preserving stable qubits is a significant hurdle with quantum computing. Qubits are highly dependent on their environment, with even minor fluctuations in temperature or electromagnetic waves interfering and actively destroying a qubit’s quantum superposition, a process called decoherence. A qubit must maintain coherence long enough to carry out meaningful computations.
Scalability: If you double the number of qubits in a quantum computer, you double the challenge of ensuring that the qubits are indeed qubits, not just classical bits. In addition to simply growing the number of qubits, we must increase our progress in reducing error rates and improving the operational fidelity of these systems. The real magic comes when these two goals are achieved—but only then.
Rate of error: QCs suffer from errors due to the fragility of qubits and breaking quantum operations. Unique quantum error correction methods are used to detect and correct errors, but they require more qubits and make the quantum circuits more complex. Finding ways to decrease mistakes without compromising efficiency and scalability is a complex problem for QCs.
Quantum Decoherence: Decoherence is a significant reason why making quantum computers is so challenging. It describes how qubits’ quantum properties are lost and become like classical bits. Finding ways to keep qubits isolated from their environment and maintain coherence for extended periods is an unanswered question in current research.
These hurdles speak to the difficulty of actually making quantum computing a reality. However, they also point to the many innovative solutions needed and their likely implementation, given further advances in quantum physics, materials science, and engineering. Such forward thinking also propels genuine optimism in quantum computing’s upcoming contribution to transformative technological changes for our future society.

Future of Quantum Computing
The coming decades of quantum computing will be full of opportunity. As one of the most radical new technologies of our day, it will face exciting (and daunting) challenges as it approaches and potentially disrupts nearly every known problem space.
Predictions: predictions of further theoretical advances in quantum computing continue to indicate that, in principle, future quantum computers could solve NP-hard problems (types of issues in which no classical computer can be proven to yield a solution in polynomial time) in seconds – equivalently, to search through tens of thousands of candidate solutions each second – whereas current classical computers would require millions of years; this includes the ability to perform quantum simulations to identify and understand the properties of large quantum systems using exponential reductions in computer time, which would ultimately lead to fundamental advancements in materials science, chemistry and physics research.
Potential effects on other industries are also enormous. In finance, optimizing trading strategies and risk management are possible, while in healthcare, the quickening of drug discovery and personalized medicine based on genetic data are promising. Logistics efficiencies ahead also include quantum computing that can optimize and globalize supply chains, thus significantly reducing costs.
Scaling quantum technologies: Building larger, more robust, and more reliable quantum computers is integral to the future of these devices. That involves improving the quality of qubits, developing sophisticated and efficient quantum error correction methods, creating scalable architectures that can support quantum algorithms, and composing many simple building blocks in complex systems.
Integration with Classical Systems: TQC will probably not replace what we mean when we say ‘classical computing.’ Instead, we will very likely see hybrid systems, those that take the best of both sides and complement the other. For example, while we cannot make elegant-looking, interacting, laser-temperature simulated electrons and protons on a classical processor, we might combine some of the best features of QP (quantum processors) and process information with them, then combine the result with the classic classical processors.
Moral and Stakeholder Concerns: Moving forward, the ethical and societal implications of quantum computing will take center stage. Issues of data privacy, security, and the digital divide will need to contend with these noble and urgent concerns before the benefits of quantum computing can be fairly distributed.
In short, we’re talking about an incredibly vibrant and dynamic space – the future of quantum computing. There’s a tremendous scientific and technological future in which quantum computers will become more practical and enable new ways of computing. We hope it will be a future where we can responsibly and equitably use this potential for the overall common good.
Quantum Computing and Cybersecurity
At the same time, quantum computing is intimately related to cybersecurity; emerging quantum technologies bring new threats and opportunities to information security.
Threats and Opportunities The coming of quantum computing is essentially a ‘two sides of the coin’ for cybersecurity: threat and opportunity. In the first instance, quantum computing coming into being will be capable of danger by potentially decoding currently available public-key encryption methods. In technical terms, though not Hilbert’s steam engine, we might imagine that it would go something like this: because a quantum computer utilizes quantum physics’ peculiar superposition, any classical process it undertakes has a potential quantum computing element. So even if some program is currently encrypted using, say, RSA or ECC (Elliptic Curve cryptography), when a few years down the line, quantum computers become powerful enough, the corresponding values and a little box of data, i.e., the key, may be vulnerable to a Schrodinger-type analysis, performed at high speed in the future quantum computer within those numbers and entering via the keyhole. After that, that’s it – nothing to stop the Borg. On the other hand, as a stroke of luck, with the instruments at hand, notably Rivest, Adleman, and Shamir’s quantum-resistant encryption algorithms, quantum computing coming into being will also provide an opportunity.
A field called quantum-safe cryptography is emerging, aiming to create encryption immune to computational attacks. Quantum key distribution (QKD) is an example that uses quantum physics to ensure that attempting to intercept a message will get picked up.
Getting ready for the Quantum Era: Might everyone, including governments worldwide and commercial enterprises, start to prepare for quantum security? Should legacy cryptographic systems be made quantum-resistant? Should more research be invested in the implications of quantum computing for global security?
Questions of ethics and law: Quantum computing would likely improve cybersecurity but raises tricky questions of ethics and law. It is already too powerful for us to control, and increasingly, we need to balance the importance of providing the tools for better, faster communications and protecting an individual’s or a nation’s privacy.
In conclusion, quantum computing presents new threats and a host of new opportunities for cybersecurity. Developing quantum-safe encryption and planning for a post-quantum world are vital to ensuring that the cybersecurity of 2050 (and beyond!) is prepared with quantum computing’s emergence in mind and that the information assets of an increasingly interconnected world will be protected and preserved.
Leading Players in Quantum Computing
The quantum computing industrial ecosystem of actors and players is fast evolving. The global tech giants and promising startups are competing to bring quantum computing technologies to market.
Corporate Giant The corporate giants are IBM, Google, Microsoft, and other Big Tech companies that are the most active in quantum computing. IBM is one of the pioneers in making their quantum computer available to the general public through various cloud services. Earlier this year, Google claimed quantum supremacy by demonstrating that a quantum processor can perform a specific task faster than any of the world’s most influential classical supercomputers. Microsoft, meanwhile, is continuing to perfect its quantum computer design while leading the efforts to develop dedicated programming languages for quantum computers.
Startups and the Quantum Future: Thanks to their small size and speed, startups can often innovate at a level that a large organization could not match. The quantum computing space is in this department, with many startups joining the quantum race. Rigetti Computing, IonQ, and D-Wave Systems are just some companies changing the quantum computing landscape through different aspects of quantum computing hardware and software, from quantum processors to quantum software and applications.
Investment Trends: The quantum computing sector is highly dynamic and richly funded, attracting funding from the public and private sectors, venture capital funds, national or state agencies, and other strategic investors. The sector’s resources are beautiful because of their disruptive potential to numerous industries.
Co-operation and Partnership: Academia, industry, and governments are collaborating to accelerate the development of quantum computing. Most significant players cooperate with leading universities and research institutes to foster innovation and develop a new workforce needed in the emerging field.
The field of leading quantum players is broad and varied. These players compete and collaborate while the branches of those players overlap and intertwine. They are all pushing the science forward to help quantum computing become a commercially viable new technology capable of worldwide implementation.

- Nature’s Quantum Computing Section
- Qiskit Foundations — Coding with Qiskit Season 1
- IBM Qiskit Summer School 2020
- Qiskit Textbook
- The Coding School’s Quantum Computing Programs
- Michael Nielsen’s Lecture Series – Quantum Computing for the Determined
- Google Quantum AI
- IBM Quantum Learning
- Quantum Game with Photons
- Introduction to Quantum Information Science by Artur Ekert
Discover more from Shadab Chow
Subscribe to get the latest posts to your email.